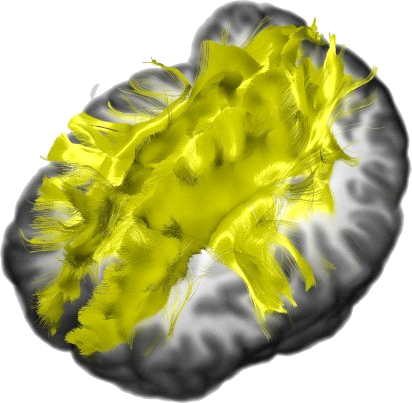
The corpus callosum, a huge band of myelinated fibers, connects the two cerebral hemispheres. Stereopsis is one mechanism for seeing depth and judging distance. Although these two features of the brain and vision are not closely related, a small minority of corpus-callosum fibers do play a small role in Stereopsis. The reason for including the two subjects in one chapter is convenience: what I will have to say in both cases relies heavily on the special crossing and lack-of-crossing of optic nerve fibers that occurs at the optic chiasm and it is easiest to think about both subjects with those anatomical peculiarities in mind.
The corpus callosum (Latin for "tough body") is by far the largest bundle of nerve fibers in the entire nervous system. Its population has been estimated at 200 million axons—the true number is probably higher, as this estimate was based on light microscopy rather than on electron microscopy—
a number to be contrasted to 1.5 million for each optic nerve and 32,000 for the auditory nerve. Its cross-sectional area is about 700 square millimeters, compared with a few square millimeters for the optic nerve. It joins the two cerebral hemispheres, along with a relatively tiny fascicle of fibers called the anterior commissure, as shown in the two illustrations on the following pages. The word commissure signifies a set of fibers connecting two homologous neural structures on opposite sides of the brain or spinal cord; thus the corpus callosum is sometimes called the great cerebral commissure.
Until about 1950 the function of the corpus callosum was a complete mystery. On rare occasions, the corpus callosum in humans is absent at birth, in a condition called agenesis of the corpus callosum. Occasionally it may be completely or partially cut by the neurosurgeon, either to treat epilepsy (thus preventing epileptic discharges that begin in one hemisphere from spreading to the other) or to make it possible to reach a very deep tumor, such as one in the pituitary gland, from above. In none of these cases had neurologists and psychiatrists found any deficiency; someone had even suggested (perhaps not seriously) that the sole function of the corpus callosum was to hold the two cerebral hemispheres together. Until the 1950s we knew little about the detailed connections of the corpus callosum. It clearly connected the two cerebral hemispheres, and on the basis of rather crude neurophysiology it was thought to join precisely corresponding cortical areas on the two sides. Even cells in the striate cortex were assumed to send axons into the corpus callosum to terminate in the exactly corresponding part of the striate cortex on the opposite side.
In 1955 Ronald Myers, a graduate student studying under psychologist Roger Sperry at the University of Chicago, did the first experiment that revealed a function for this immense bundle of fibers. Myers trained cats in a box containing two side-by-side screens onto which he could project images, for example a circle onto one screen and a square onto the other. He taught a cat to press its nose against the screen with the circle, in preference to the one with the square, by rewarding correct responses with food and punishing mistakes mildly by sounding an unpleasantly loud buzzer and pulling the cat back from the screen gently but firmly. By this method the cat could be brought to a fairly consistent performance in a few thousand trials. (Cats learn slowly; a pigeon will learn a similar task in tens to hundreds of trials, and we humans can learn simply by being told. This seems a bit odd, given that a cat's brain is many times the size of a pigeon's. So much for the sizes of brains.)
Not surprisingly, Myers' cats could master such a task just as fast if one eye was closed by a mask. Again not surprisingly, if a task such as choosing a triangle or a square was learned with the left eye alone and then tested with the right eye alone, performance was just as good. This seems not particularly impressive, since we too can easily do such a task. The reason it is easy must be related to the anatomy. Each hemisphere receives input from both eyes, and as we saw in Chapter 4, a large proportion of cells in area 17 receive input from both eyes. Myers now made things more interesting by surgically cutting the optic chiasm in half, by a fore-and-aft cut in the midline, thus severing the crossing fibers but leaving the uncrossed ones intact—a procedure that takes some surgical skill. Thus the left eye was attached only to the left hemisphere and the right eye to the right hemisphere. The idea now was to teach the cat through the left eye and test it with the right eye: if it performed correctly, the information necessarily would have crossed from the left hemisphere to the right through the only route known, the corpus callosum. Myers did the experiment: he cut the chiasm longitudinally, trained the cat through one eye, and tested it through the other—and the cat still succeeded. Finally, he repeated the experiment in an animal whose chiasm and corpus callosum had both been surgically divided. The cat now failed. Thus he established, at long last, that the callosum actually could do something—although we would hardly suppose that its sole purpose was to allow the few people or animals with divided optic chiasms to perform with one eye after learning a task with the other.
Physiology
One of the first neurophysiological examinations of the corpus callosum was made a few years after Myers' experiments by David Whitteridge, then in Edinburgh. Whitteridge realized that for a band of nerve fibers to join homologous, mirror-symmetric parts of area 17 made no sense. No reason could possibly exist for wanting a cell in the left hemisphere, concerned with points somewhere out in the right field of vision, to be connected to a cell on the other side, concerned with points equally far out in the left field. To check this further Whitteridge surgically severed the optic tract on the right side, just behind the optic chiasm, thus detaching the right occipital lobe from the outside world—except, of course, for any input that area might receive from the left occipital lobe via the corpus callosum, as you can see from the illustration on this page. He then looked for responses by shining light in the eyes and recording from the right hemisphere with wire electrodes placed on the cortical surface. He did record responses, but the electrical waves he observed appeared only at the inner border of area 17, a region that gets its visual input from a long, narrow, vertical strip bisecting the visual field: when he used smaller spots of light, they produced responses only when they were flashed in parts of the visual field at or near the vertical midline. Cooling the cortex on the opposite side, thus temporarily putting it out of commission, abolished the responses, as did cooling the corpus callosum. Clearly, the corpus callosum could not be joining all of area 17 on the two sides, but just a small part subserving the vertical midline of the visual field.
Anatomical experiments had already suggested such a result. Only the parts of area 17 very close to the border between areas 17 and 18 sent axons across to the other side, and these seemed to end, for the most part, in area 18, close to its border with area 17. If we assume that the input the cortex gets from the geniculates is strictly from contralateral visual fields—left field to right cortex and right field to left cortex—the presence of corpus-callosum connections between hemispheres should result in one hemisphere's receiving input from more than one-half the visual fields: the connections should produce an overlap in the visual-field territories feeding into the two hemispheres. That is, in fact, what we find. Two electrodes, one in each hemisphere near the 17-18 borders, frequently record cells whose fields overlap by several degrees.
Torsten Wiesel and I soon made microelectrode recordings directly from the part of the corpus callosum containing visual fibers, the most posterior portion. We found that nearly all the fibers that we could activate by visual stimuli responded exactly like ordinary cells of area 17, with simple or complex properties, selective for orientation and responding usually to both eyes. They all had receptive fields lying very close to the vertical midline, either below, above, or in the center of gaze, as shown in the diagram on this page.
Perhaps the most esthetically pleasing neurophysiological demonstration of corpus-callosum function came from the work ofGiovanni Berlucchi and Giacomo Rizzolatti in Pisa in 1968. Having cut the optic chiasm along the midline, they made recordings from area 17, close to the 17-18 border on the right side, and looked for cells that could be driven binocularly. Obviously any binocular cell in the visual cortex on the right side must receive input from the right eye directly (via the geniculate) and from the left eye by way of the left hemisphere and corpus callosum. Each binocular receptive field spanned the vertical midline, with the part to the left responding to the right eye and the part to the right responding to the left eye. Other properties, including orientation selectivity, were identical, as shown in the illustration on the facing page.
This result showed clearly that one function of the corpus callosum is to connect cells so that their fields can span the midline. It therefore cements together the two halves of the visual world. To imagine this more vividly, suppose that our cortex had originally been constructed out of one piece instead of being subdivided into two hemispheres; area 17 would then be one large plate, mapping the entire visual field. Neighboring cells would of course be richly interconnected, so as to produce the various response properties, including movement responses and orientation selectivity. Now suppose a dictareally happened, since the brain had two hemispheres long before the cerebral cortex evolved.
This experiment ofBerlucchi and Rizzolatti provides the most vivid example I know of the remarkable specificity of neural connections. The cell illustrated on this page, and presumably a million other callosally connected cells like it, derives a single orientation selectivity both through local connections to nearby cells and through connections coming from a region of cortex in the other hemisphere, several inches away, from cells with the same orientation selectivity and immediately adjacent receptive-field positions—to say nothing of all the other matching attributes, such as direction selectivity, end-stopping, and degree of complexity. Every callosally connected cell in the visual cortex must get its input from cells in the opposite hemisphere with exactly matching properties. We have all kinds of evidence for such selective connectivity in the nervous system, but I can think of none that is so beautifully direct.
Visual fibers such as these make up only a small proportion of callosal fibers.
In the somatosensory system, anatomical axon-transport studies, similar to the radioactive-amino-acid eye injections described in earlier chapters, show that the corpus callosum similarly connects areas of cortex that are activated by skin or joint receptors near the midline of the body, on the trunk, back, or face, but does not connect regions concerned with the extremities, the feet and hands.
Every cortical area is connected to several or many other cortical areas on the same side. For example, the primary visual cortex is connected to area 18 (visual area 2), to the medial temporal area (MT), to visual area 4, and to one or two others. Often a given area also projects to several areas in the opposite hemisphere through the callosum or, in some few cases, by the anterior commissure. We can therefore view these commissural connections simply as one special kind of cortico-cortico connection. A moment's thought tells us these links must exist: if I tell you that my left hand is cold or that I see something to my left, I am using my cortical speech area, which is located in several small regions in my left hemisphere, to formulate the words. (This may not be true, because I am left handed.) But the information concerning my left field of vision or left hand feeds into my right hemisphere: it must therefore cross over to the speech area if I am going to talk about it. The crossing takes place in the corpus callosum. In a series of studies beginning in the early i96os, Roger Sperry, now at Cal Tech, and his colleagues showed that a human whose corpus callosum had been cut (to treat epilepsy) could no longer talk about events that had entered through the right hemisphere. These subjects provided a mine of new information on various kinds of cortical function, including thought and consciousness.